Investigating Energy Solutions for the Petrochemical Industry
August 02, 2024
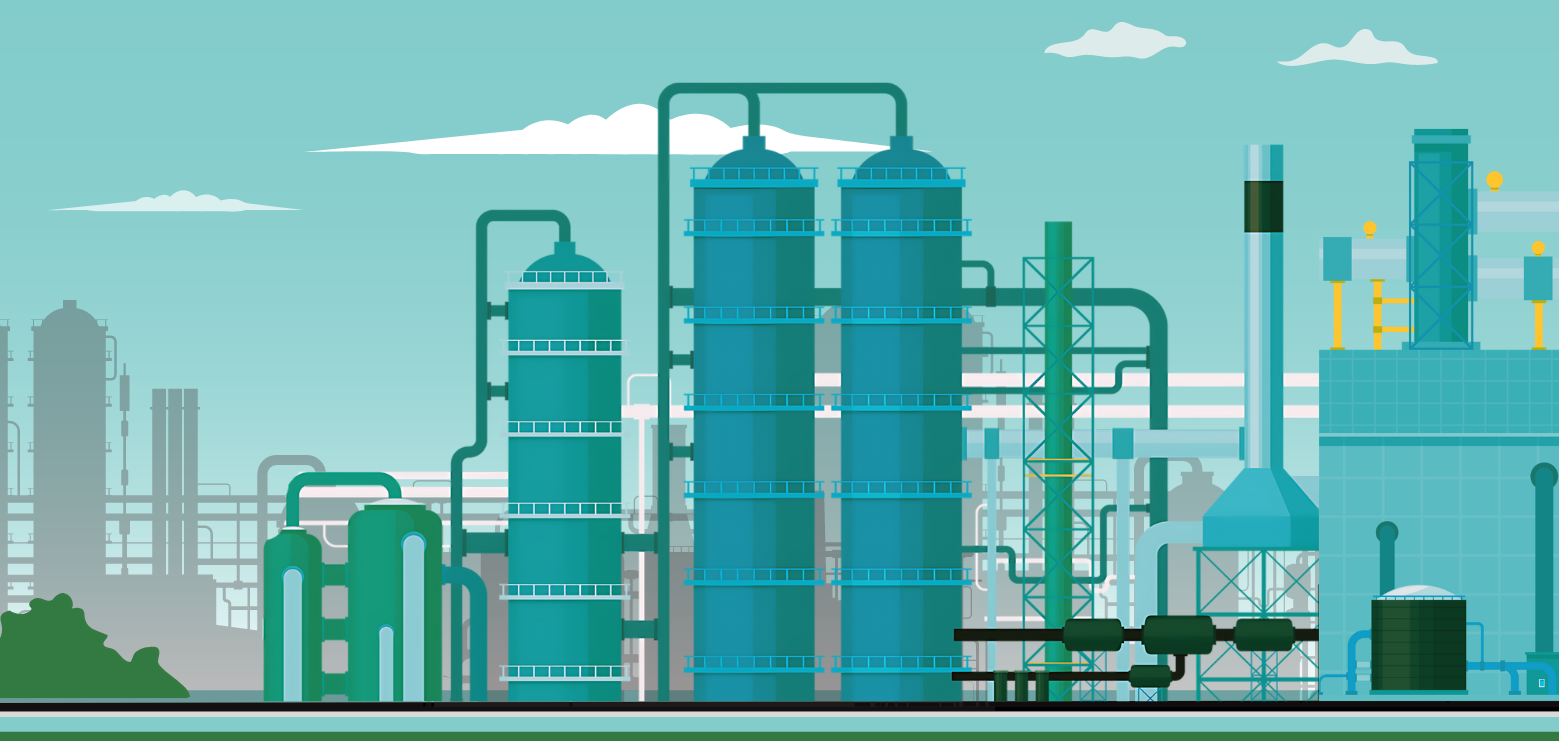
The chemical industry ranks among the largest energy consumers in the United States, relying heavily on energy for processing, fuel production, and facility operations to develop chemicals that address critical challenges in food supply, health, and materials. Louisiana exemplifies this trend, with its total energy consumption ranking third overall and first per capita, primarily due to its energy-intensive chemical, petroleum, and natural gas industries.1
Assistant Professor Matthew Chambers from the LSU Department of Chemistry is working to change this trend. Through innovative techniques and industry collaboration, Chambers is investigating methods for the petrochemical industry to produce high-value commodities more energy-efficiently, thereby reducing the environmental impact.
“In a more energy- and environmentally conscious society in which we need to exist, we must revisit our reliance on high temperature, high-pressure processes that are not environmentally friendly but are often utilized by the chemical industry by necessity,” Chambers stated.
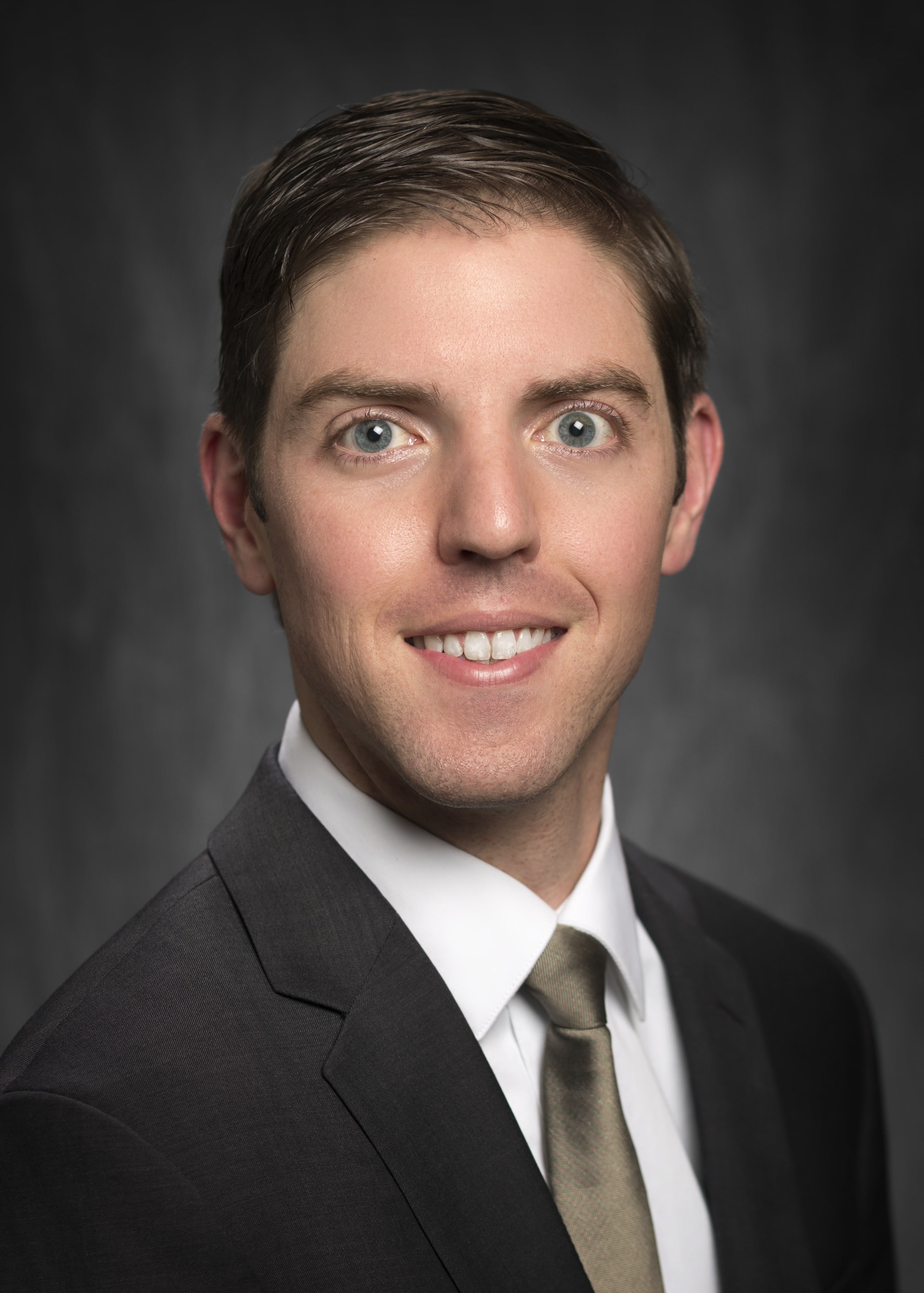
Assistant Professor Matthew Chambers, LSU Department of Chemistry
In 2021, Chambers was awarded funding by the Louisiana Board of Regents Industrial Ties Research Subprogram (ITRS), which fosters faculty research and promotes state economic development through collaborations between academic programs and industrial and business entities. In partnership with Shell Global Solutions US, Inc., Chambers is investigating the process of hydroformylation, one of the highest-volume homogeneously catalyzed industrial processes used today that uses large amounts of energy.
Hydroformylation is a pivotal chemical reaction in which aldehydes are produced from alkenes, carbon monoxide, and hydrogen gas. The process involves adding a formyl group to an alkene and is catalyzed by transition metals like rhodium or cobalt at high temperatures and pressures. The resulting aldehydes serve as versatile intermediates for synthesizing various chemicals and products, such as coatings, adhesives, and detergents.
While rhodium-based catalysts can operate at relatively low temperatures and pressures, their low availability and price limit widespread usage. Cobalt, on the other hand, is a cheaper and more abundant metal but demands more extreme operating conditions, thus potentially consuming more energy. Access to the feed tolerance and robustness of high-pressure systems under milder conditions with a cobalt catalyst has been a long-standing challenge with hydroformylation.
In a recent paper published in the Journal of the American Chemical Society, or JACS, the Chambers group evaluated a cationic cobalt(II) hydroformylation pre-catalyst, introduced by other scientists in 2020 as a potential medium-pressure hydroformylation technology.2
“There’s a lot of controversy behind this pre-catalyst,” Chambers noted. “Before this system, every cobalt-based catalyst has involved a 1+ charge on the metal. It may sound subtle, but this new system has a 2+ on the cobalt center. This changes everything about the potential catalyst, including how many things it can interact with and how it interacts with those compounds. It also was reported to be indefinitely stable! With such excitement, others tried to develop this pre-catalyst further but achieved inconsistent results. The entire efficacy of the pre-catalyst to mediate hydroformylation was being disputed. We identified that a limiting factor was a robust characterization method to confirm the nature and purity of the pre-catalyst at the start.”
Past studies of this cobalt pre-catalyst used Nuclear Magnetic Resonance, or NMR, to investigate its characteristics, which left room for error since the resonances were broad and difficult to measure due to the paramagnetic nature of Co(II). Determined to resolve these discrepancies, the Chambers group sought assistance from the LSU Mass Spectrometry Facility, working with facility director Fabrizio Donnarumma.
Utilizing the ESI-TOF Mass Spectrometer, the Chambers group characterized the pre-catalyst, unveiling new discoveries. The once-thought-stable pre-catalyst was found to have significant air sensitivity, causing rapid compound decomposition. However, the group also discovered that solvent serves as a fifth coordination site on the cobalt center, opening the door for a tunable site. Shell Global Solutions will now evaluate the pre-catalyst using larger-scale industrial-relevant conditions.
My group is committed to advancing this research with the long-term objective of developing energy-efficient processes for the petrochemical industry, paving the way for a more sustainable future.
Matthew Chambers, Assistant Professor of Chemistry
The Chambers group is also investigating carbon-hydrogen, or C–H, functionalization, another process by which petrochemical companies produce high-value chemicals. This process involves transforming C–H bonds into carbon-heteroatom or carbon-carbon bonds.
Traditionally, C–H functionalization has been a multi-step process requiring introducing and removing functional groups, often resulting in energy inefficiencies and higher costs. However, with funding from an NSF CAREER award, Chambers aims to advance this process by developing more direct modifications of less reactive C–H bonds using photochemistry techniques.
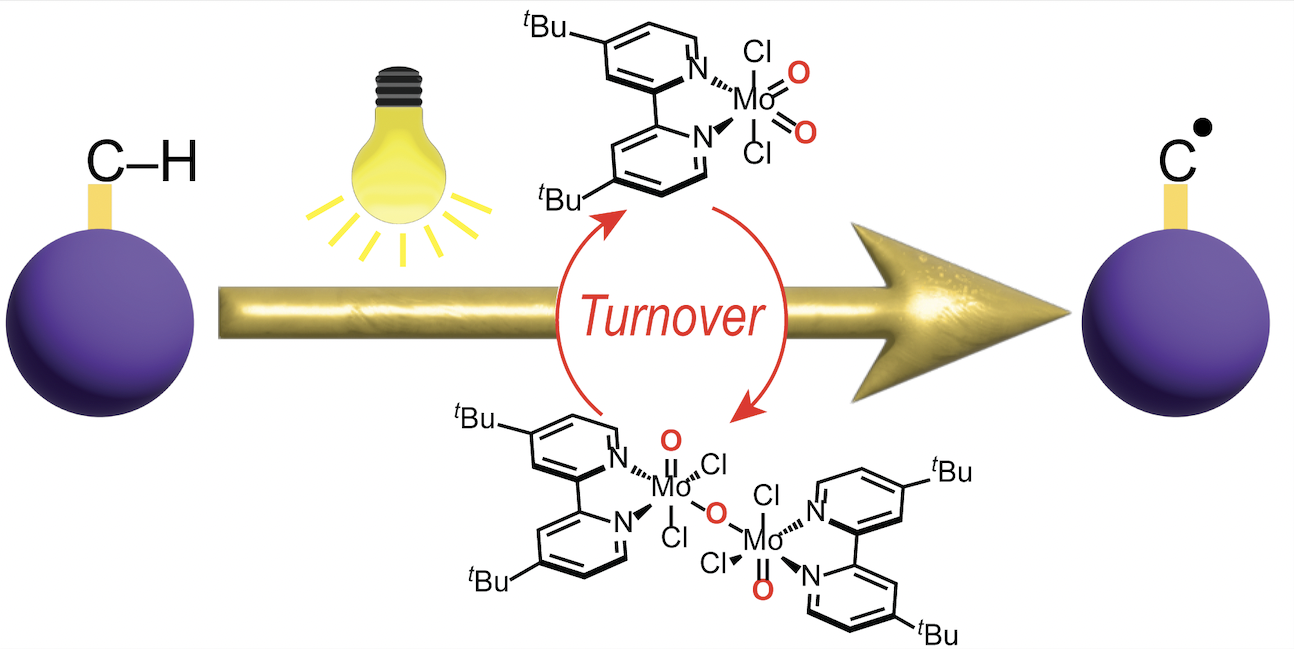
Direct photocatalytic C–H activation mediated by MoO2Cl2(bpy-tBu), a unique photoactive metal OXO. [Baumberger, et al. (2024). Chem. Commun., 60, 6901.]
“Photochemistry has the potential to harness light energy for more efficient and sustainable chemical transformations,” Chambers stated. “Specifically, we are exploring the photochemical reactivity of metal-oxo complexes to enhance catalysis and alter selectivity. Using light to drive these reactions opens the possibility of converting abundant petrochemical resources into commodity feedstocks with less environmental impact.”
The Chambers group reported one of the first examples of a transition metal oxo complex that can directly mediate C–H bond activation photocatalytically.3 Leveraging a molybdenum VI dioxo platform, the group demonstrated that hydrocarbon activation readily occurs in the presence of sacrificial oxidants and exposure to light.
Although the study did reveal that the production of H₂O during the reaction limits photocatalyst stability, the reported findings provide critical insights into developing sustainable methods using light for hydrocarbon conversion.
The Chambers group's published findings were recognized in the themed collections for 2024 Emerging Investigators and Chemical Communications HOT Articles 2024. Their work represents a significant step in investigating sustainable chemical processes with potential innovative solutions to address the petrochemical industry's long-standing energy consumption challenges.
- U.S. Energy Information Administration. Louisiana: Profile Overview. Accessed July 15, 2024.
- Holzknecht, D. R., Van Alstine, A. K., Russell, B. P., Vinyard, D. J., Donnarumma, F., & Chambers, M. B. (2024). Revisiting the preparation and catalytic performance of a Phosphine-Modified CO(II) hydroformylation precatalyst. Journal of the American Chemical Society.
- Baumberger, C. L., Valley, V. Z., & Chambers, M. B. (2024). Direct photocatalytic C–H functionalization mediated by a molybdenum dioxo complex. Chemical Communications.