Nuclear Physics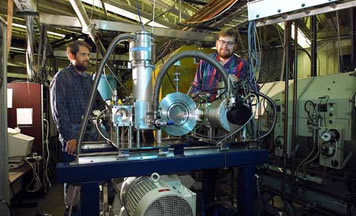
Faculty:
Atomic nuclei are fundamental to studies ranging from quarks to cosmology. Nuclei are the laboratories in which we study the smallest constituents of matter (quarks and gluons) and the fundamental symmetries of nature (parity and time-reversal invariance). Relativistic collisions between nuclei test our ideas of fundamental forces, such as quantum chromodynamics (QCD) under extreme conditions, and help us glean glimpses of what the universe must have been like a few second after the Big Bang. Nuclear reactions powered the stars and forged the elements during the Big Bang and supernova explosions. Finally, by exploiting nuclear reactions to detect astrophysical particles such as neutrinos, cosmic rays, and dark matter, physicists have a powerful probe to "look" into the inner working of the universe.
The excitement of modern nuclear physics is its interdisciplinary nature and the use of a wide range of techniques and tools. LSU faculty members are users of two major nuclear research facilities, the Holifield Radioactive Beam Facility at Oak Ridge National Laboratory and the Isotope Separator and Accelerator at TRIUMF. Their research work probes frontiers in nuclear structure physics, nuclear astrophysics, and nucleosynthesis. Work being carried out at the Jefferson Lab National Accelerator Facility enables other members of the faculty to gain a better understanding the structure of nuclei through linkage to the substructure of their constitutent nucleons.
LSU has both experimental and theoretical research groups in nuclear physics, with strong concentrations with the high-energy physics and astrophysics/space science groups. The principal foci of the experimental group is the study of nuclei far from stability and relativistic heavy-ion collisions, while the principal theme of the theory group is in the area of low-energy nuclear structure, bridging from nuclear dynamics to quark/gluon constituents and to astrophysical/cosmology applications.
The structure of the atomic nuclei represents a unique challenge to a nuclear theorist. The number of constituent particles is greater than two, yet far less than Avogadro's number, so neither exact quantum mechanical solutions nor statistical methods can be widely applied. There is not an obvious force center as in atoms nor a lattice, as often applies in solid state physics. While the essential features of the nucleon-nucleon interaction can be deduced from scattering experiments, how it gets renormalized in the nuclear medium is not well understood. Many nuclei can be described in macroscopic terms as rotating and vibrating spheroids, but many others require a microscopic shell-model interpretation. Because of all of these complications, nuclear theory is a computationally intensive arena.
The goal of the nuclear theory group is to provide an overall theoretical understanding of the structure and reactions of atomic nuclei at a fundamental level and predict properties of light and medium-mass nuclei that are key to pinpointing the origin, structure, and evolution of baryonic matter in the Universe. This is achieved by recognizing the symmetries that govern the nuclear dynamics and by employing state-of-the-art shell models with modern nucleon-nucleon interactions. This brings together techniques in many-body physics, group theory, and high-performance computing. Some of the research themes are an ab-inito (from first principles) description of light nuclei, a microscopic description of phenomena ranging from pairing to clustering correlations and collective rotations, symmetry-based approaches for nuclear reactions and projectile-target interactions, the limits of nuclear stability, nuclear astrophysics, and the weak interaction of nuclei.